The most astounding fact about the universe
Back in 2008, Time Magazine interviewed Neil de Grasse Tyson, and asked him, “What is the most astounding fact you can share with us about the Universe?” His answer was indeed a very good, true, and astounding fact about the Universe: that all the complex atoms that make up everything we know owe their origins to ancient, exploded stars, dating back billions of years. It’s a great fact, and it’s definitely on the short list of the most remarkable things we’ve learned about the Universe.
But if I were to choose the single most astounding fact about the Universe, I’d want you to consider something else: something far more fundamental and profound. Consider that the Universe — with everything in it the way it is — didn’t have to be this way. It didn’t have to even be close.
We could have had a Universe without trees, without mountains, without our skies and without oceans. We could have had a Universe without planets like Earth, or planets at all. We could have even had a Universe where nothing that we know of — no particles, forces or interactions — exists as it does right now.
Yet despite the endless possibilities for what could have been, this is the Universe we have. Our Universe exists the way it is, with all the particles, forces, interactions, structures, and the unique history of how it all came to be. The way it all turned out, no doubt, is absolutely wondrous. Just here, in our own little corner of the Universe, we find ourselves in a forgotten, nondescript little group of galaxies no more or less special than any of the billions out there.
But while our planet, our galaxy and our place in the Universe might not be special or privileged in any fundamental way, the Universe itself, compared to all the ways it could have been, is something very special. It manifests itself on every scale we can conceive of looking at. We can look all the way down to the smallest scales, to the internal structure of matter, down to molecules, atoms, and the most fundamental subatomic particles ever discovered.
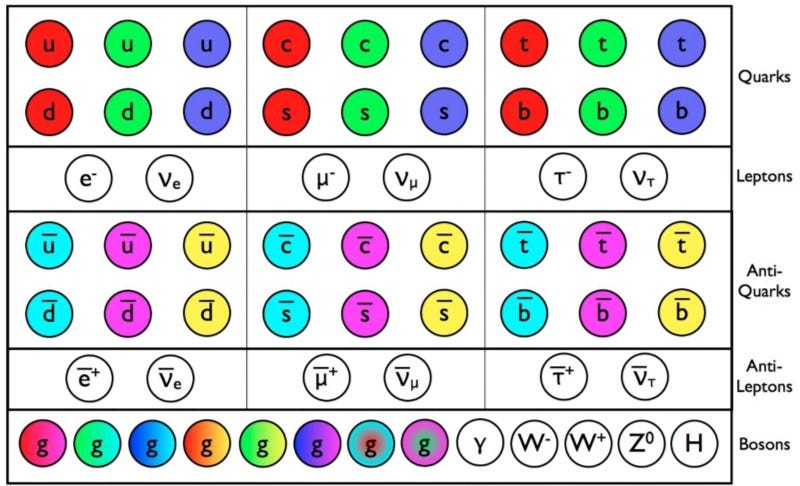
Image credit: E. Siegel, from his new book, Beyond The Galaxy.
We can look out, not just to the stars and galaxies, but all the way across the Universe, to quasars, intergalactic clouds of gas, even back to the cosmic microwave background and the very first neutral atoms our Universe had ever seen. We can look at all of that, and everything in between.
- on all scales,
- in all places,
- and at all times,
From the weakest, lowest-frequency photon of light to the largest galaxy ever assembled, from the unstable atoms of Uranium decaying in the Earth’s core to the neutral hydrogen atoms forming for the first time 46 billion light years away, the laws that everything in this Universe obeys are the same.
Gravitation, electromagnetism, and the strong and weak nuclear forces are the same wherever and whenever you go. The particles that exist (and can exist) and their properties are the same. The rules that govern the entire system are the same. All of it, at all energies, at all times, at all places, are underwritten by the same laws of nature.
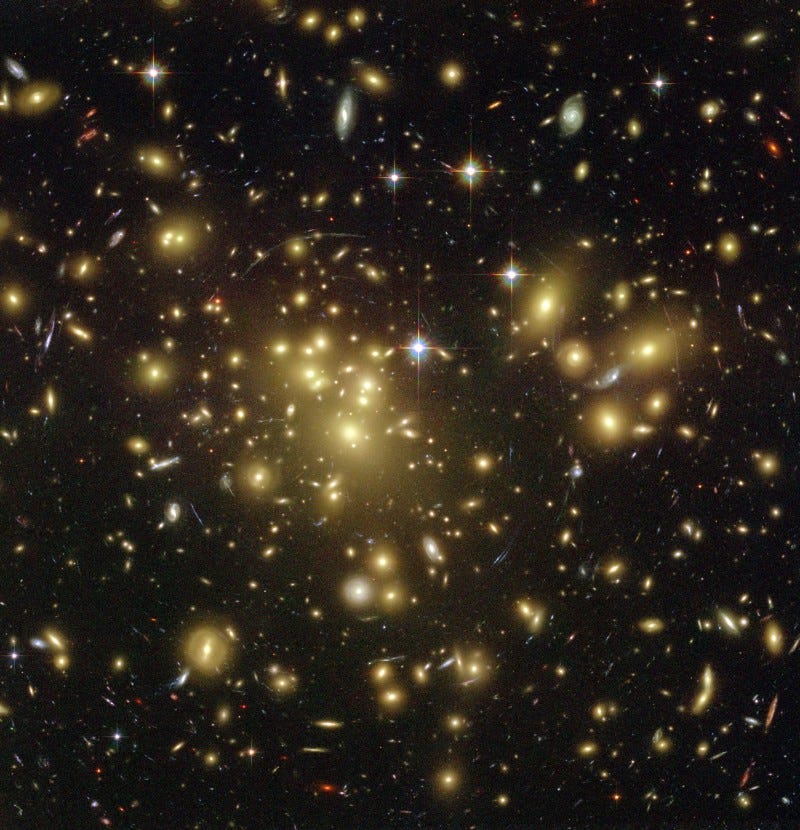
Image credit: NASA, ESA, the ACS Science Team and N. Benitez et al.
This is the most remarkable thing of all. Imagine what things would be like if this weren’t true. Imagine an existence where nature behaves randomly and unpredictably, where gravity turns on-and-off on a whim, where the Sun could simply stop burning its fuel for no apparent reason, where the atoms that form you could spontaneously cease to hold together.
A Universe like this would truly be frightening, because it could never be understood. The things you learn here and now might not be true later, or even five feet away. But the Universe isn’t like this at all.
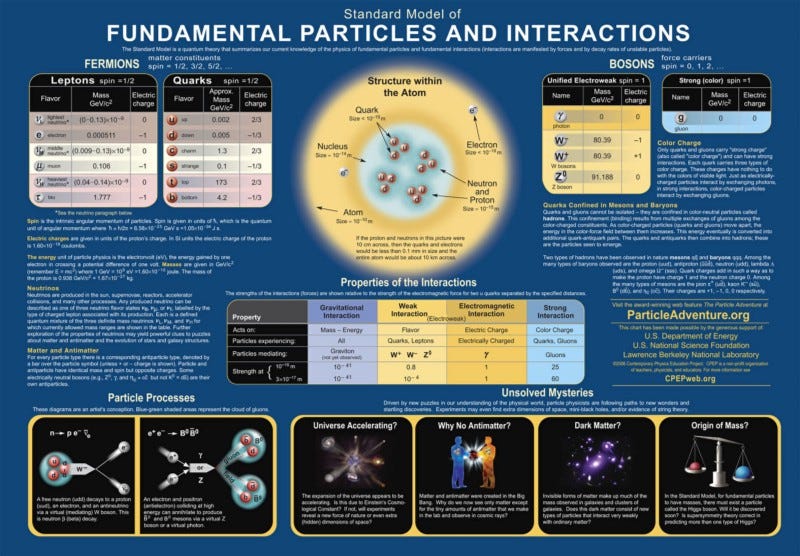
Image credit: U.S. DOE, NSF, CPEP and LBNL, retrieved from http://physics.gla.ac.uk/.
The Universe is a place where the forms that the matter and energy occupying it can change, where the spacetime itself that we all exist in can change, but the fundamental laws — that everything is subject to — are constant.
Because what that means is that we can observe the Universe, experiment with the Universe, assemble and disassemble the things we find in it, and learn about the Universe itself.
Only if the fundamental laws of the Universe are the same everywhere and at all times can we learn what they actually are today. Only if those laws are applicable everywhere and at all times can we use that knowledge to figure out what the Universe — and everything in it — was doing in the past, and what it will be doing in the future.
In other words, it is this one fact, this most astounding fact, that allows us to do science, and to learn something meaningful, at all. It’s why any form of science even exists, and why it’s actually a useful tool for learning about this Universe. When you put it all together, it means the most astounding fact about the Universe is this: that it exists in such a way that it can be understood at all.
This post first appeared at Forbes and then at 3tags.org
Source: 3tags.org