The biggest question about the beginning of the universe
Our Universe is expanding, getting less dense and cooling today, teaching us that it was hotter and denser in the distant past. If we extrapolate backwards in time, we can reach epochs where:
- gravitation hadn’t yet had time to collapse matter into clusters, galaxies or even stars,
- the temperature of the Universe was too hot to form neutral atoms, ionizing them immediately,
- particles were so energetic that even atomic nuclei were unstable, being immediately split apart into individual protons and neutrons,
- and even to where the energy density was so high that matter/antimatter pairs were spontaneously created from pure energy.
Above is the earliest known “baby picture” of our Universe. When the Universe finally did cool enough to stably form neutral atoms, all the radiation from the earliest times could suddenly travel through space, in a straight line, without being absorbed, re-emitted or scattered off of a free, charged particle. This radiation then had its wavelength stretched by the expansion of the Universe, where it can now be found at microwave frequencies: the Cosmic Microwave Background (CMB), or the leftover glow from the Big Bang. When we look at the fluctuations in it — or the slight imperfections from a perfectly uniform temperature at various locations across the sky — we can use what we know about physics and astrophysics to teach us a number of very important things.
One of the things we can learn is that our Universe is made up of about 5% normal (atomic) matter, 27% dark matter and 68% dark energy. But no less important is this: we learn that these imperfections were initially the same on all scales, and are of such a small magnitude that the Universe couldn’t have achieved an arbitrarily high temperature in the distant past. Instead, there must have been a phase before the Universe was hot, dense and matter-and-radiation filled that set it all up. Originally conceived by Alan Guth in 1979, this phase — known today as cosmic inflation — solves a number of major problems with the Universe: stretching it flat, giving it the same temperature everywhere, eliminating high-energy relics and defects (like magnetic monopoles) from the Universe, and providing a mechanism to generate those much-needed fluctuations.
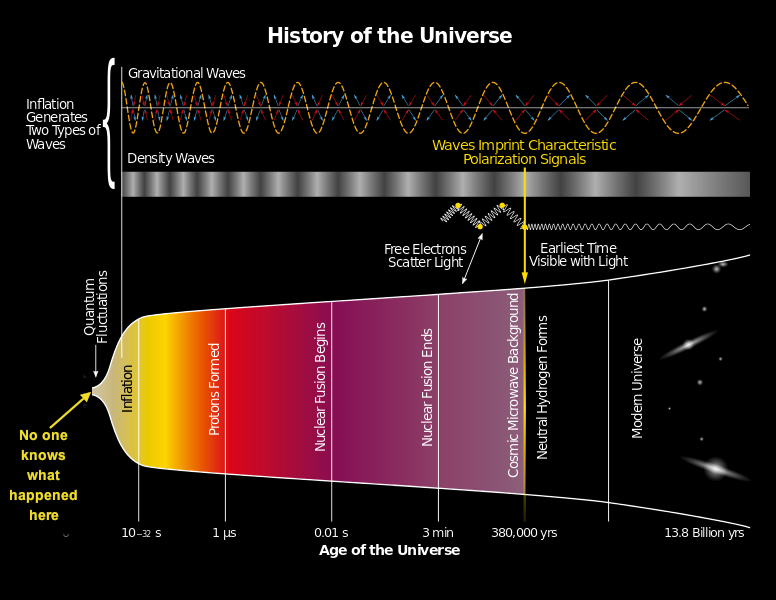
- The fluctuations can be more important on small scales or on large ones, and by measuring the full spectrum of them, we can know what the slope of that hill was when inflation came to an end.
- If we can measure the gravitational wave fluctuations and compare them to the density fluctuations, we can reconstruct how the slope was changing when inflation ended.
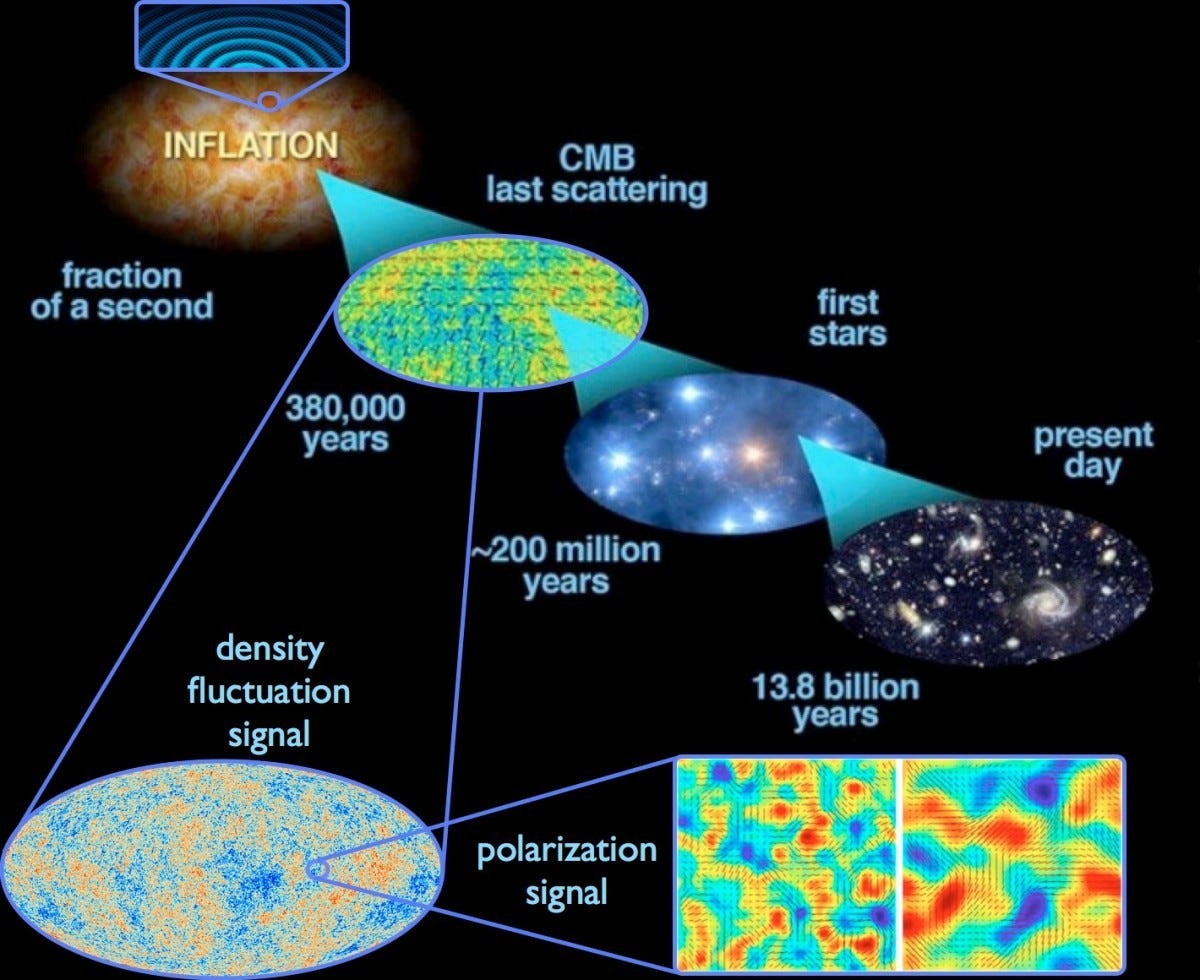
Thanks to the Planck spacecraft, we now have very tight restrictions on the density fluctuations, disfavoring many of the simplest models. As superior (polarization) data from projects like Planck, BICEP, POLARBEAR and others continues to come in, hope that we’ll either detect the gravitational wave signatures or set stronger limits than ever before rises even higher. People have argued for a long time that cosmic inflation has too many solutions, but the better we get at making these measurements, the more hope we have that the number of solutions will eventually be reduced to one unique one.
The Universe has a great story to tell us about its origin, to the limits of what we can conceivably measure. The better we get at actually making those measurements, the better we can understand how it all got its start. Cosmic inflation is almost definitely the answer to what happened before the Big Bang. But what was cosmic inflation like? We’re closer than ever to actually coming up with the answer.
Sources:3tags.org; startswithabang
Image credits: NASA; E. Siegel
No comments:
Post a Comment